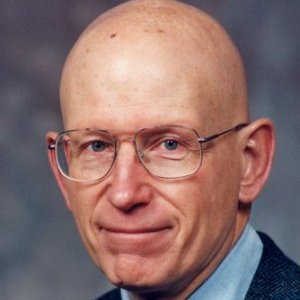
Tom Swiontek, Ph.D.
Professor Emeritus Milwaukee School of Engineering
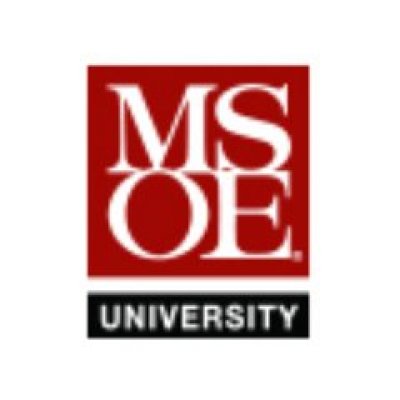
Milwaukee School of Engineering
View more experts managed by Milwaukee School of Engineering
Education, Licensure and Certification
Ph.D.
Electrical Engineering
Marquette University
1975
B.S.
Electrical Engineering
Marquette University
1969
Biography
He has written a number of publications, and is a member of the Institute of Electrical and Electronic Engineers and the American Society for Engineering Education.
Areas of Expertise
Accomplishments
Bacon Fellowship
NSF Traineeship
Oscar Werwath Distinguished Teacher Award, MSOE
1998
Biomedical Engr. Scholastic Achievement Award
Graduate Cum Laude
Affiliations
- Institute of Electrical and Electronics Engineers (IEEE) : Member
- American Society for Engineering Education (ASEE) : Member
- Tau Beta Pi : Member
Research Grants
Undergraduate Design Project to Aid the Disabled
NSF Grant
Principal Investigator
Selected Publications
A mixed-signal EEG interface circuit for use in first year electronics courses
IEEELee, V., Monski, J., Williams, W., Muthuswamy, B., Swiontek, T., Maharbiz, M., Subramanian, V., Kovac, F.
2012-06-01
In their first electronics course, many students find operational amplifiers, analog filters and sensor interface circuitry perplexing and daunting. The purpose of this paper is to present a circuit that addresses these pitfalls. A simplified electroencephalogram (EEG) circuit that is interfaced to a digital backend is proposed. The completed circuit involves using instrumentation amplifiers and filters for the EEG interface. The digital backend helps analyze EEG data on the computer.
Electric Field Distribution Within Normal Cat Spinal Cord
Journal of NeurotraumaKhan, T., Myklebust, J., Swiontek, T., Sayers, S.
1994-06-01
Electric currents of small magnitude have been used successfully to induce regrowth of injured spinal cord fibers. The purpose of this study was to determine the potentials and current density distributions on the surface, as well as within the spinal cord, after the application of exogenous electric fields. A 10 μA DC current was applied epidurally to the spinal cord using two different electrode configurations. The two electrode configurations studied were: anode and cathode dorsal (D-D) and anode ventral and cathode dorsal (V-D). Two types of recording electrodes were used to map the potentials on the surface and within the spinal cord. The recording system consisted of glass microelectrodes connected to differential amplifiers. The output was recorded on a polygraph. The current density was more localized on the dorsal surface of the spinal cord for the D-D configuration. In contrast, in the V-D configuration, the current density was greater near the anode on the ventral surface and near the cathode on the dorsal surface of the spinal cord. As a result of the anode being located ventrally, there was a more uniform current density distribution within the spinal cord.
Electrical Field Distribution within the Injured Cat Spinal Cord: Injury Potentials and Field Distribution
Journal of NeurotraumaKhan, T., Myklebust, J., Swiontek, T., Sayers, S., Dauzvardis, M.
1994-06-01
This study investigated the spontaneous injury potentials measured after contusion or transection injury to the cat spinal cord. In addition, the distribution of electrical field potentials on the surface and within the spinal cord were measured following applied electrical fields after transection and contusion injuries. After transection of the spinal cord, the injury potentials were −19.8 ± 2.6 mV; after contusion of the spinal cord, the injury potentials were −9.5 ± 2.2 mV. These potentials returned to control values within 2.5–4 h after injury. The electrical field distribution measured on the dorsal surface, as well as within the spinal cord, after the application of a 10 μA current, showed little difference between contusion and transection injuries. Scalar potential fields were measured using two configurations of stimulating electrodes: dorsal to dorsal (D-D), in which both electrodes were placed epidurally on the dorsal surface of the spinal cord, and ventral to dorsal (V-D), in which one electrode was placed dorsally and one ventrally. As reported in normal uninjured cats, the total current in the midsagittal plane for the D-D configuration was largely confined to the dorsal portion of the spinal cord; with the V-D configuration, the current distribution was uniform throughout the spinal cord. In the injured spinal cord, the equipotential lines midway between the stimulating electrodes have a wider separation than in the uninjured spinal cord. Because the magnitude of the electrical field E is equal to the current density J multiplied by the resistivity r, this suggests that either the current density is reduced or that the resistivity is reduced.
A Probe for Measuring Current Density During Magnetic Stimulation
Biomedical Instrumentation & TechnologyTay, G., Chilbert, M.A., Battocletti, J., Sances, J.A. and Swiontek, T.
1991-06-03
Time-varying magnetic fields induce currents in conductive media, and when the induced current is large enough in excitable tissue, stimulation occurs. This phenomenon has been applied to the human brain and peripheral nerves for diagnostic evaluation of the neural system. One important aspect that is presently unknown is the current level necessary in tissue for stimulation induced by magnetic fields. This study presents a method of measuring the induced current density from pulsed magnetic fields in vitro and in vivo. The current-density probe was inserted into three concentrations of saline and into the brains of ten anesthetized cats. Two stimulation systems with coils 9 cm and 5 cm in diameter were used. The two systems provided sinusoidal and pulsatile coil currents. Measurements made in saline were compared with those calculated theoretically for a semi-infinite medium. The measured values were within 5% of the calculated values. Measurements made in the cat brain showed a 67% decrease compared with the theoretic model. This variance is attributed to the finite bounds of the skull. The results indicate that direct measurement of current density is possible. Subsequent measurements will aid in the design of improved magnetic stimulation systems.
Measurement of current density induced in cat brain during transcranial magnetic Stimulation
Journal of Clinical EngineeringChilbert, M., Tay, G., Battocletti, J., Sances, A., Swiontek, T., Maiman, S., Suh J. U.
1992-06-01
This study measures in vivo current densities induced in the brains of fifteen cats during transcranial magnetic stimulation. This wasessential for determining regions of excitation. Three magnetic stimulators were used in this study. Two of the stimulators were constructedin-house; one used a 5-cm diameter coil that was driven with a damped sinusoidal signal; the other used the same coil and was drivenwith an undamped sinusoidal signal. The damped system was operated at a frequency of 620 Hz and damping time constant of 0.912 ms.It generated a peak magnetic field of 140 gauss at the center of the coil. The undamped system was operated at a frequency of 3 KHz andgenerated a peak magnetic field of 28 gauss. The third system is a Cadwell MES-10 stimulator. Results showed that current distributionpatterns of the laboratory-made stimulators were similar. Thus, a coil driven with a damped sinusoidal input induces the same currentdistribution as when it is driven with an undamped sinusoid. Also, current density induced in the tissue was found to be maximum near theedge of the coil and decreased as the edge of the coil moved away from the region of interest. This corresponds to mathematical modelspreviously developed (Guidi, 1989; Ferguson, 1989; Roth, 1990; Reuter, 1988). Inducing maximum current at a region, however, does notnecessarily imply tissue stimulation in that particular region. Neurophysiologic factors, such as nerve fiber diameter and orientation,infuence neural excitability. Thus, it is necessary to consider these parameters during magnetic stimulation.